
The way we look at material use is changing. Minimising material use in construction needs to, and should, be of environmental, commercial and aesthetic benefit to projects. We are moving from assessing material usage based on the construction, to assessing over the design life of the project and to ecological time frames. We will move towards assessments in geological time frames. London Structures Lab is committed to exploring how these shifts in understanding should impact structural design.
Our seven steps to minimising material usage have been linked the RIBA project stages, highlighting the significance of early decisions, and to enable the progression from philosophical overview to practical application within projects. The guidance is intended to aid reflection of future stages whilst in the earlier stages.
The Status Quo
The MEICON report (https://www.meicon.net/survey2018) produced by Cambridge University includes some damning statistics for UK structural engineering consultants. Key findings of the report for the industry are:
· Average material usage of less than 50% of capacity (source Monihan, M. and Allwood https://royalsocietypublishing.org/doi/pdf/10.1098/rspa.2014.0170)
· An average of 35-45% of the steel used in typical steel framed office buildings, by mass, is not required for structural efficiency
· Potential to reduce material usage in concrete structures by 30-40% through design optimisation.
· 63% of beams dominated by serviceability requirements rather than strength requirements
We all know the current pressure on natural resources and the impact that it is having on the planet. Many in the sector are already signatories of either Architects Declare or Engineers Declare. Why, with increasing levels of awareness, do shocking levels of inefficiency persist?
The declaration states that signatories will share data and be open with best practice, these are essential to the evolution in the industry.
The MEICON survey did not identify a single leading factor but did show that the barriers to greater material efficiency are both cultural and technical. Both areas need to be addressed but whilst the technical can be seen as the domain of the engineer, cultural factors are due to industry wide norms that must be addressed collectively.
Landsecurities’ Lavington Street development is an early leader in a collective approach to material usage. It has a maximum CO2 per square meter specified in the design brief for all parties to work to. Steps like this are enablers to widespread industry change but require method of measurement to be shared to create standard processes.
Here at London Structures Lab we believe the first question should always be “do we need a new building?” Can we improve our existing stock to provide greater utilisation, either through improved occupation levels or through building reinvention.
Assuming it has been determined a new building is required, here are our seven suggested steps for industry professionals to adopt to achieve long term reductions in material usage:
1. Establish the right brief (RIBA Stage 0/1)
Light-weighting, long-life loose-fit or design for de-constructability? Debate about establishing the right design strategy for a building should be fundamental to the design process. Reconsidering permanence on a holistic, zonal and component basis is an integral part of any progressive design brief.
A lightweight design solution is considered to be the default design if the question of design life is not raised in the brief. However, actively pursuing lean design is very different from continuing with the status quo, as can be seen from the assessment of the current construction norms. To truly design light-weight the structural system has to challenge the traditional approaches and standard performance criteria. Looking at a holistic strategy, such as integrated structural and façade systems or profiled structural elements is essential to achieving minimum material usage. This often leads to a highly specific building, which potentially limits the life span of the structure but reduces the energy that goes into producing it to a minimum.
Long life-loose fit introduces adaptability into a structures design to ensure that it is capable of enabling changes of use. It is not simply increasing the loading capacity of the structure, though this may be a factor to consider, evolution of use rarely has implications for structural loadings. It has to acknowledge the buildings use, or elements of the building, will evolve over time. This might affect strategic thinking – for example, decoupling of the structural stability system from the service core, to anticipate that risers and lifts might change as MEP systems evolve. Though this requires more embodied energy the longer lifespan created reduces the per year assessment.
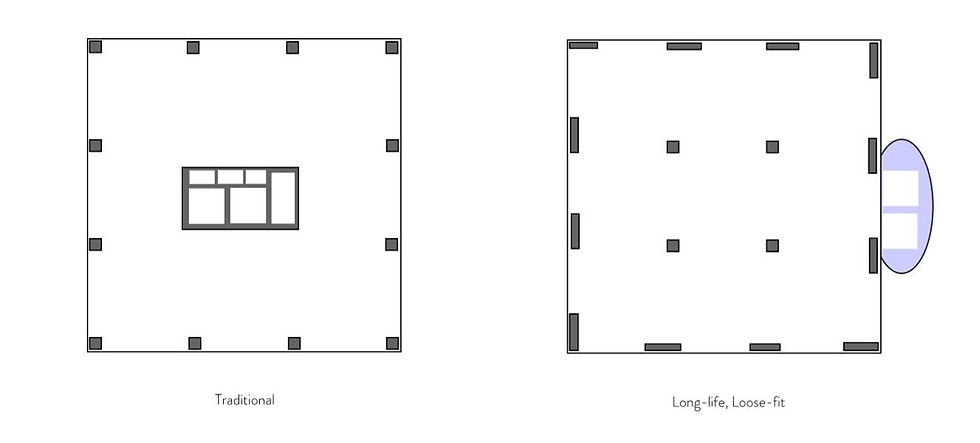
A third and arguably the most important structural strategy in this context is to design for deconstructability, enabling materials to enter the circular economy. There is some precedence of this occurring with steel framed structures- such as the University of Toronto, which recovered 16 tonnes of steel from a nearby demolition- but the current barriers are significant. When it comes to the re-use of components that will be subject to the demolition process, it is challenging to provide suitable warrantees for material that may have lost its QA trail. To circumvent this engineers consider a lower specification for reused material, limiting its effectiveness. What we should be doing is designing structure to be de-mountable and using BIM systems to track material in use, recording its certifications and enabling it to be used again to its full capacity. Sensing and material passports would facilitate this and can be coupled with in-situ monitoring of stresses that can record loading history. Designing buildings for de-mountability now and designing future buildings around component re-use is the most energy efficient and potentially cost effective strategy- if we do not increase the CO2 required to build it. Increasing the embodied energy of construction to give a capability for demounting that is never used would not be the right solution, and accepting that some areas of a building might not change is challenging to determine. It takes long term vision to implement this, not least because of the requirement to store and track materials to facilitate their reuse.
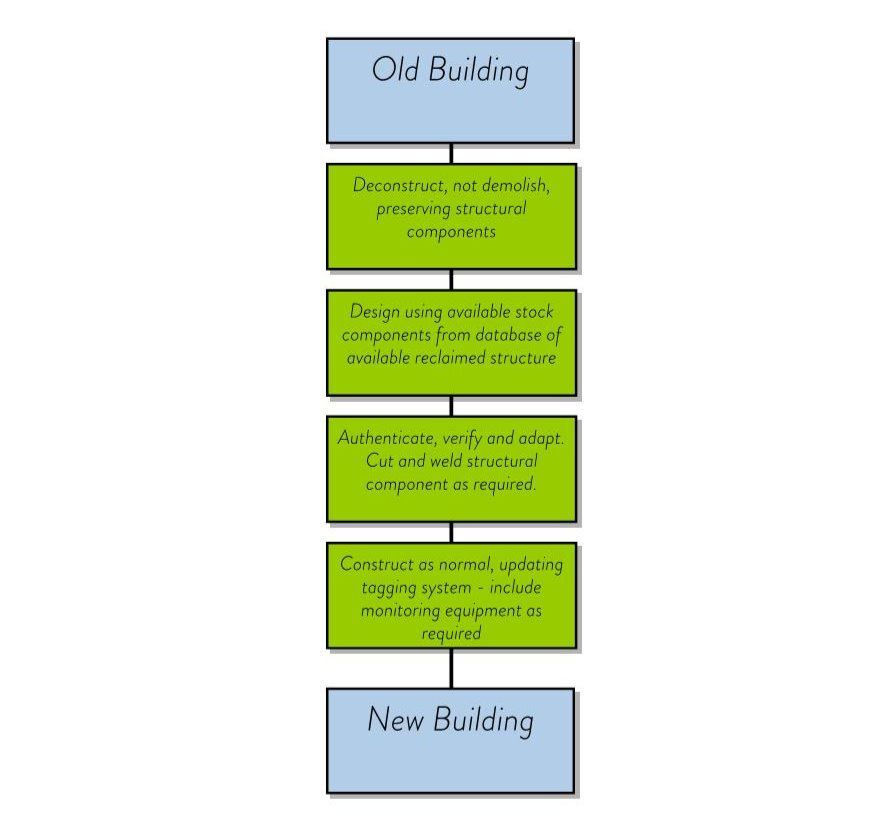
At the outset of a project a discussion on the buildings’ design life and strategy should be held, considering all three approaches and their application to different areas of the scheme. Considering the initial design to major refurbishment, as well to demolition (and potentially reuse) enables cost effective, material efficient solutions to evolve in the subsequent design phases. It unlocks conversations about varying levels of durability between components of the build, potentially allowing for the renewal of façade and cores, whilst retaining the majority of the primary structure. This process of assessment should reduce both immediate and whole life costs for the development.
2. Challenge design parameters (RIBA Stage 2)
Structural loadings for many buildings are defined prior to the design for the interior being completed. It is widely appreciated by many engineers that most buildings are unlikely to ever realise the full design loadings, but design loadings reflect a solution that “works” providing flexibility in the selection of fit out choice and building use. As the context we design in changes, over design needs to be questioned, through monitoring of existing structures and reviewing the parameters of design.
Serviceability limits for design generally relate to movement and acceleration of structures, as opposed to structural failure through material failure. Movement limitations are primarily governed by follow on trades, such as the header details for fire stopping, or the sensitivity of the façade system. Their influence on the quantum of materials in a structure is significant and their necessity is not always clear at the initial stages of a project. Interaction with the suppliers for follow on trades on the impact of increased allowable movement can enable a reduction in structural materials. In our experience the influence of structural movement on the detailing of the follow on trade is often misunderstood. Similarly dynamic accelerations and their effect on occupancy are under-developed- see the VSimulator research by the Universities of Bath and Exeter for more details on how this is being addressed https://vsimulators.co.uk/
When developing design options it should be clear what overall brief is being followed, what design parameters are being used and how they relate to follow on trades should all be clearly stated. Coupling this information with carbon trackers (discussed further in item 6 below) enables informed decisions to be made by the collective team.
3. Adjust the attitude to risk (RIBA Stage 2)
Design to 100% utilisation. Design codes provide methodologies for calculating the minimum performance requirements for a structural element and already include safety factors. The inclusion of such a a requirement to design to 100% utilisation within codified or other design guidance has not affected current attitudes to risk and the level of design development at the point of fixing the structural design solution.
One of the key factors quoted in the MEICON survey as reason for designing to 80% of capacity as opposed to 100% was design uncertainty (https://www.meicon.net/uncertainty). This isn’t an issue that can be solely being addressed by structural engineers, the design process can ensure that once clarity is achieved on structural performance parameters the project enables the design to be revisited.
As an industry we want finite answers. At the early stages of design, what could be offered is a range of structural sizes depending on how the design develops. As this impacts everything from design detailing to net areas it would take a significant, industry-wide change to adopt, but it allows the refinement of the structural aspects of the design to match the level of design development. Ultimately this should give opportunity for an optimised design.
4. Embrace technological progression (RIBA Stage 3)
Once the design is clarified, and fundamental decisions such as building geometry, occupational organisation, principle materials and structural principles are confirmed a greater level of detail can be studied.
Technical innovation in fabrication is developing, as is the link between analysis and fabrication. How can future designs be informed by the manufacturing advances? Automation of the fabrication process should enable more specific links between the performance parameters defined by the analysis and the material usage in production. Profiled beams and slabs have to date been the province of academic research and one-off structures. Wide scale implementation needs to address the industry drivers of standardisation. Whilst standardisation is a less efficient use of materials it is not a flippant choice, it enables faster production and construction programs and simplification of interaction with other aspects of the building. The progress in capability of tracking material from its raw state, through the fabrication process and to its in-use location should facilitate a greater level of specificity in design. Closer correlation between analysis and fabrication has been stagnated by issues of information responsibility. Contractual procedures need to adapt to the capability of technology to enable this to be unlocked.

Offsite manufacture frequently reports significant reduction in construction waste to only 1%, with typical on-site construction having 20% material wastage- see the Get it Right report for more information https://getitright.uk.com/. The open source platforms, discussed in London Structures Lab article on DfMA, are one way to create stronger links between the materials used and the material requirements.
5. Analyse real building performance (RIBA Stage 3/4)
As most buildings have at least some bespoke aspects to their design there are factors influencing structural behaviour which frequently go unaccounted for or concealed within a design envelope of potential behaviours. There is an assumption that analytical assessments will accurately represent structural behaviour.
Structural analysis is frequently element based, but real behaviour assessment requires system based assessments, where adjacent elements are included in the analysis so the interaction between them can be assessed. For example, steel frames are frequently designed assuming rotational flexibility at connections, which is unlikely to reflect true behaviours. Particularly due to the temporary stability requirements that steel frame erection has, which are likely to mean that end plate connections are required for multi-storey systems, where fin plates are frequently assumed for the permanent system. Unlike many other disciplines, structural engineers tend not to utilise mock-ups and testing to justify their design assumptions. Within developments with repetitive structural systems, train stations and airports being an obvious example where benefits could be realised. This requires this work to be incorporated into the design program and budget. In-situ tagging and monitoring would also facilitate monitoring the nature of real building performance to a greater level of detail.
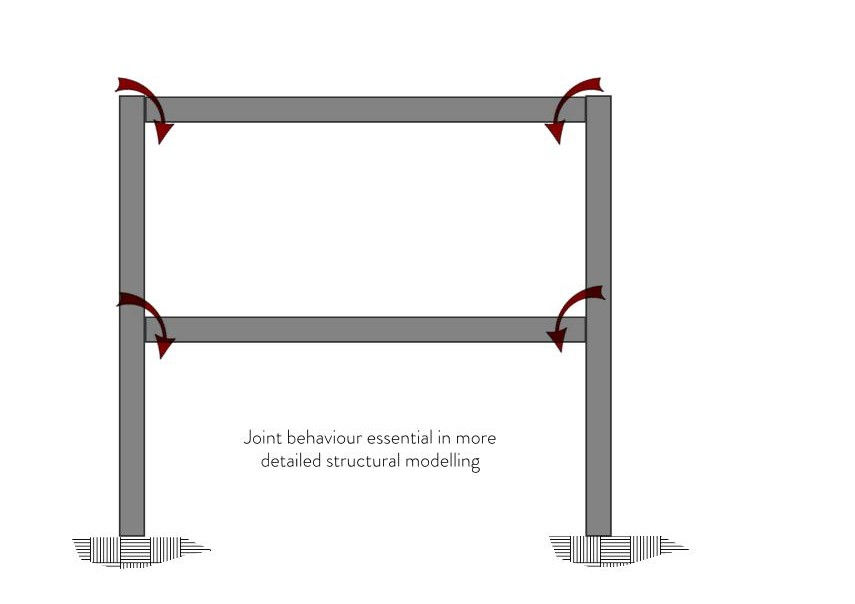
Engineers can produce a map of the real load capacity of their floors. It is standard practice to include design loadings in an operations manual for the building. Including real capacity would facilitate future developments in how the building is occupied, or how it is redeveloped/ reinvented.
6. Measure and re-assess (RIBA Stage 4)
The basic tools to assess carbon content are available from the Environmental Agency and facilitate the early assessment of options studies against carbon usage. You can download it here: https://data.gov.uk › data › contracts-finder-archive › download
As the specifications for the contract are being written significant steps can be made to ensure that lower energy products are being used. However, the parameters for this are constantly evolving, as steel production starts to use cleaner energy sources and as concrete innovation enables the reduction of cement content. It’s never popular to unpick a design at this stage of the program, but feeding back data from contractor tenders (which you will have to request) into the Stage 2 options updates the awareness of the team as to the impact of the decisions made. It also facilitates progression in assessments of future projects.
7. Monitor buildings in use (RIBA Stage 7)
Post occupancy monitoring is seldom performed in structural engineering but is essential in the ratification of any significant overhaul of the design guidance. This should include monitoring of building usage against design loads to see if further reduction to cumulative live loading is viable- potentially reducing the sizing of the vertical structure at the lower levels of multi-storey buildings- is sensible. It would also include monitoring of building behaviour to determine whether dynamic responses and structural deflections are being accurately assessed during the analysis stages. This feedback loop enables the progression of design guidance to reflect changing occupancy habits and progression in specification and analysis.

With this step, as with most of the actions outlined above, the sharing of data is key to making any significant advancements. Moving towards a data driven approach to design requires data. It sounds simple, but this is one of the key stumbling blocks preventing structural engineering being the progressive discipline it should be.
Recommended further reading:
Comments